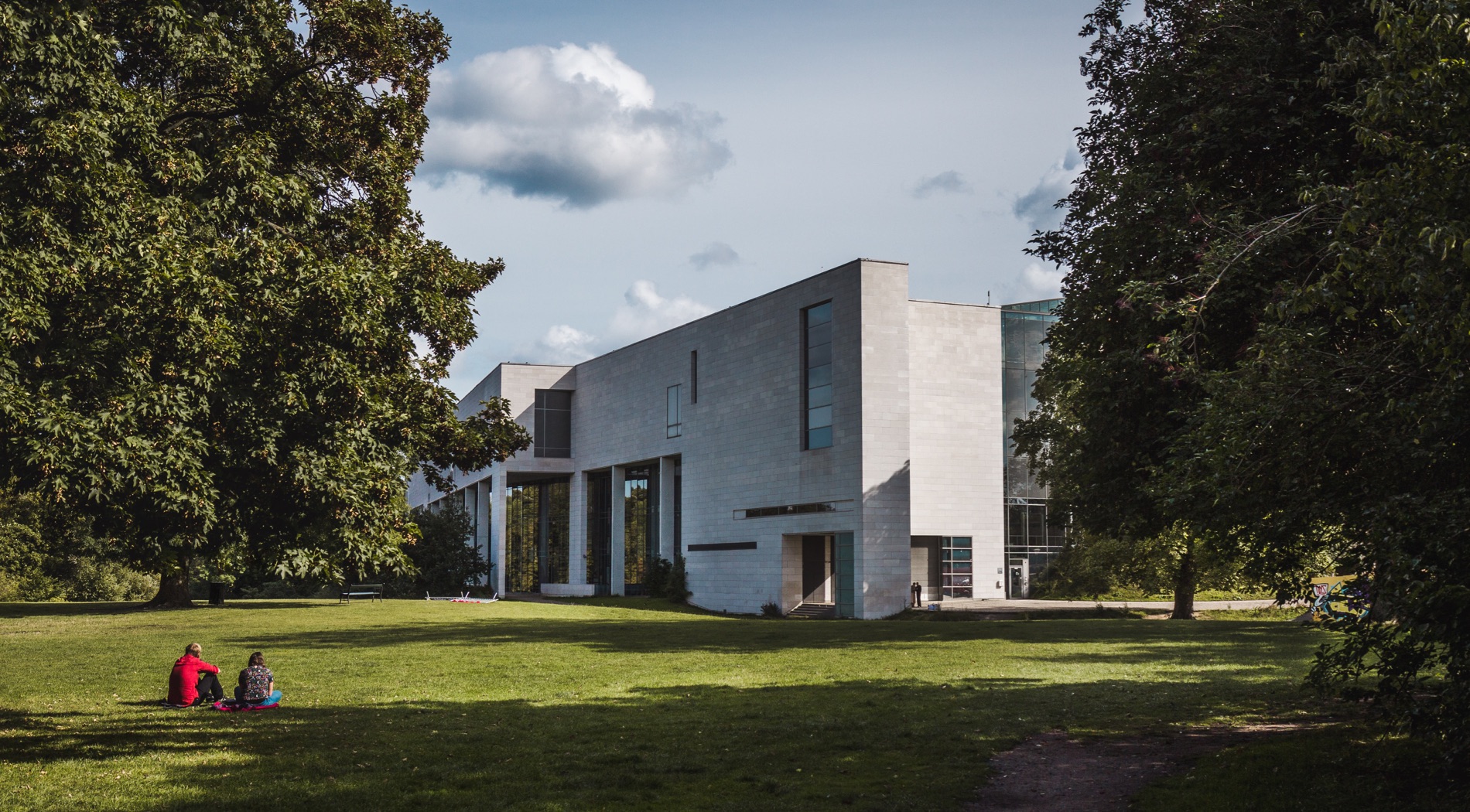
Content used with permission from ARCHITECT Magazine and Architecture 2030. Originally published January 15, 2020.
To promote his designs for lightweight structures, Buckminster Fuller famously asked, “How much does your building weigh?” Today, as architects realize that both structural efficiency and carbon efficiency are necessary, the question has become, “How much carbon does your building embody?”
Many architects are pushing for the reduction or elimination of embodied carbon emissions, as is already happening with operational energy. Beginning in 2020, for example, global architecture and engineering firm HOK plans to conduct a life cycle assessment on the structures of all of its new whole-building projects, and to “look for opportunities to optimize our specifications,” says Anica Landreneau, Assoc. AIA, director of sustainable design.
Indeed, the building structure and sub-structure are good places to hunt because, together, they constitute more than half of a commercial building’s embodied carbon footprint. Moreover, architects and developers should look for opportunities to reuse and renew existing structures, so as not to waste the energy already expended (and carbon dioxide already emitted) to create them. Architecture 2030’s Carbon Smart Materials Palette and the Embodied Carbon in Construction Calculator, or EC3, a tool developed by the Carbon Leadership Forum, C Change Labs, and Skanska, are great places to investigate the impact of material selection for both retrofit and new construction projects.
In weighing the options of steel, concrete, or wood, factors to consider include project location, scale, expected service life, reuse potential, and even the material metrics being compared (see “How to Measure Embodied Carbon"). As awareness of embodied carbon increases, industries are angling to make their products more appealing. Architects alone can’t reduce the embodied carbon of their projects, but they are the party most likely to have the client’s ear, and can affect the supply chain through their specifications. Before finalizing material decisions, leverage the increasingly user-friendly comparison tools and bring builders and suppliers into the conversation—the earlier, the better.
Steel
The global steel sector has a massive carbon footprint, contributing upwards of 10% of global carbon dioxide emissions. “The major steel companies are very well aware of the demands of society to reduce emissions, and they are looking for ways to respond to that,” says Matthew Wenban-Smith, executive director of ResponsibleSteel, an international, not-for-profit organization based in Wollongong, New South Wales, Australia.
Manufacturing virgin steel from iron ore is energy intensive. Basic oxygen furnaces (BOFs), the norm in much of the developing world, require coke—a purified version of coal—to extract the iron from the ore and alloy it with carbon. The result, pig iron, is then refined into mild steel, which incorporates about 25% recycled iron and steel scrap. Manufacturers are experimenting with ways to replace coal and coke with non-fossil agents like hydrogen and electrolysis in BOF mills.Source: EPD InternationalSample Environmental Product Declaration (EPD) template.
The American Institute of Steel Construction estimates that 98% of structural steel from demolished buildings is recovered and recycled into new steel products. As such, domestically produced structural steel, which comes from electric arc furnace (EAF) mills, boasts a recycled content of 93%, according to a UL-verified Environmental Product Declaration (EPD) authored by the AISC in 2016. “A car door, a steel beam, a shipping container, or an old refrigerator could be sold as scrap and turned into a steel wide-flange beam that goes into a new skyscraper,” says AISC adviser Luke Johnson.
Because EAF mills run on electricity, they largely are as green as their power source. Last September, a 19th-century Colorado steel mill owned by Russian conglomerate Evraz reached a deal with the local power utility to build an adjacent 240-megawatt solar array, which will supply a significant portion of the mill’s energy. Steelmaker Nucor is building a $250 million mill in Missouri that will be entirely powered by wind. Other, similar projects are in the works.
ResponsibleSteel recently published the first version of its eponymous standard, a voluntary, international benchmark designed to support “the responsible sourcing and production of steel” and developed through a multi-stakeholder process involving manufacturers, like ArcelorMittal, and environmental advocacy groups, like Mighty Earth. Certification is based upon third-party auditing and independent panel approval.
Wenban-Smith, who helped draft sustainability standards in forestry prior to focusing on steel, wants to make steel one of the world’s cleanest materials: “When the energy footprint for EAF becomes neutral, we can think of steel in a fully circular economy,” he says. Realizing that dream, if possible, would likely occur first in developed nations, where large quantities of scrap are available for recycling.
What to ask when specifying steel
- Is steel available from an electric arc furnace mill, and, in particular, one that is powered by renewable energy?
- Can the structure use braced frames instead of moment-resisting frames to reduce the mass of steel required?
- Can you source ResponsibleSteel-certified steel?
Concrete
People love to hate concrete almost as much as they love using it. As the world’s most ubiquitous construction material, it contributes 6% to 11% of global carbon dioxide emissions. Most of those emissions come from the production of its binder, portland cement, which comprises about 10% of the concrete mix by weight, on average. Producing aggregate—sand and crushed rock, which may constitute 70% to 80% of the mix, on average—also requires energy, but much less so. Sand mining can damage river and coastal ecosystems.
Nearly half of cement’s carbon dioxide emissions results from the burning of fossil fuel to heat cement kilns to about 2,500 F. Advances in clean energy production can help reduce these emissions: Solar-powered cement plant prototypes in California and France by Heliogen and Solpart, respectively, have successfully heated kilns to around 1,800 F using enormous mirror arrays. However, the majority of carbon dioxide emissions come from chemical reactions that are inherent to cement’s production: In a process called calcination, limestone breaks down into carbon dioxide—which escapes into the atmosphere—and quicklime, an ingredient of cement.
Some companies have sequestered carbon dioxide into concrete, including Dartmouth, Canada–based CarbonCure and Los Gatos, Calif.–based Blue Planet. These processes remain the exception and, to date, do not fully offset the carbon emissions of concrete production.
Currently, the most straightforward way to reduce concrete’s carbon footprint is to use less cement, as the Carbon Smart Materials Palette makes clear. So-called blended cements use uncalcined limestone and other supplementary cementitious materials (SCMs) in place of a portion of clinker—the hard, kiln-formed pellets that are ground and mixed with other ingredients—in the manufacture of cement. Encompassing natural pozzolans, such as rice husk ash, and industrial byproducts, such as fly ash, blast-furnace slag, and silica fume, SCMs can improve the structural performance of concrete as well as lower its embodied carbon.
North America lags behind Europe in adopting blended cements, says Julie Buffenbarger, senior scientist and sustainability principal at Mendota Heights, Minn.–based Beton Consulting Engineers. Specifications are a good way to make concrete construction more efficient, she notes, but being too prescriptive can be counterproductive. Rather than dictate the ingredient proportions in a concrete mix, she suggests design teams specify performance criteria in terms of measurable plastic and hardened properties, “so the producer can provide options within the constraints.” Keep in mind that SCMs may extend the times needed to reach the required strength benchmarks.
What to ask when specifying concrete
- Can the amount of cement be reduced in the concrete mix?
- Can the overall mass of concrete in the project be reduced?
- What is the least energy-intensive cement kiln that is locally available?
- What carbon-sequestering aggregate or mix techniques can be included?
Timber
Innovations in mass timber, notably the domestic production of engineered products such as cross-, glue-, nail-, and dowel-laminated timber, have fueled hopes of making carbon-neutral building attainable on a large scale. According to industry-backed analyses, the trees felled—and replaced—to produce mass timber can sequester more carbon during their growth than what’s emitted to manufacture, transport, and construct with it. Increased demand for sustainably sourced timber could incentivize reforestation. “We have to re-cover parts of this planet with forests,” HOK’s Landreneau says. “We can impact that by specifying wood construction where possible.”
Engineered wood panels can replace steel decking and concrete floor slabs in commercial structures, and glulam columns can take the load of mid-rise and high-rise structures from their steel and concrete counterparts. These timber products can also be fire-resistant and quick to assemble. “The day they’re installed and connected to the structural frame, they’re able to carry their full design load,” says Kenneth Bland, a vice president at the American Wood Council.
But does mass timber live up to its billing as environmentally sustainable?
According to the U.S. Department of Agriculture, the total volume of trees growing in U.S. forests has increased 60% since 1953. As demand for engineered wood products ramps up, forests that produce mass timber will have to be managed sustainably, with trees replanted after harvesting. Differences in forest management practices translate into wide variations in the amount of carbon sequestered, so knowing where and how your timber products were made is important.
More research is needed on the carbon emissions of the logging, processing, and transport of wood products. New industry-average EPDs for wood and timber products are expected in the first quarter of 2020, but declarations from specific wood manufacturers would be even more useful. Beyond the carbon embodied in the wood itself are emissions from soil and forest impacts, and from the manufacture of the resins and glues that bind mass timber layers.
Finally, mass timber’s carbon storage advantage only holds true if the beams and panels remain in service or are prevented from decomposing, which would release carbon back into the atmosphere. To be durable, wood structures must be protected from water, insect, or fungal infiltration potentially through the use of sealants combined with the sound design and detailing of the building envelope. To be reusable, timber members should be joined by removable fasteners.
What to ask when specifying wood
- Is your wood product reclaimed? If not, does it come from a sustainably managed forest?
- Can the structure be assembled with removable fasteners to allow for potential reuse of the members?
- Does the project use efficient framing techniques that minimize the mass of the timber required?
The Blueprint for Better campaign is a call to action. AIA is asking architects, design professionals, civic leaders, and the public in every community to join our efforts. Help us transform the day-to-day practice of architecture to achieve a zero-carbon, resilient, healthy, just and equitable built environment.